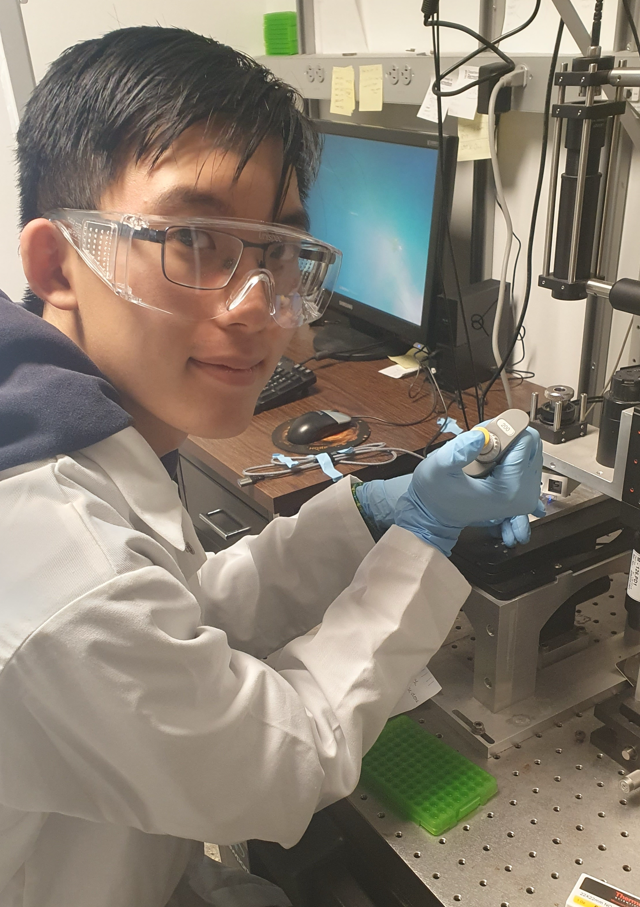
Hydrogels are crosslinked and flexible networks of polymer chains with high water content. Depending on their material properties, hydrogels have a variety of biomedical, electronic, and structural applications, such as for contact lenses and drug delivery. The bulk properties of a hydrogel, such as ductility, ability to self-heal, and light responsiveness, can be tuned by introducing different polymeric crosslinks into the network. Peptoids—biocompatible, synthetic, and sequence-defined polymers analogous to biological peptides—are one such crosslinker candidate. Peptoids can be designed to have a degree of self-attraction, and therefore different portions of the polymer can bind to one another (effectively shortening the polymer extension) or unbind (lengthening the polymer). These bonds can be reversibly broken by applying stretching forces onto the peptoids, which could allow for the desired rearrangement of polymers in a hydrogel. The characterization of these peptoid conformations is therefore of great interest in order to motivate the design of novel composite hydrogel crosslinkers, allowing for well understood and tunable local plastic response by the hydrogel. For this project, we synthesize peptoid hairpin loops with a charge-neutral central region between two oppositely charged or hydrophobic domains, enabling the polymer to self-bind. We then carry out precision single-molecule elasticity measurements on these peptoid hairpins using magnetic tweezers to gain insight into their conformational response to external forces. Conducting force-extension measurements for a variety of peptoid sequences, lengths, and solvent conditions, we then compare peptoid linkage dynamics to better understand the effects of sequence on chain conformation. Furthermore, if multiple linkages between the charged peptoid regions unbind in unison, we could potentially observe cooperative unfolding behavior in these synthetic copolymers, as is common in biopolymers but has proved challenging to replicate in synthetic systems.